Quantum Computing Has Far Greater Promise Than Just Nuclear Security
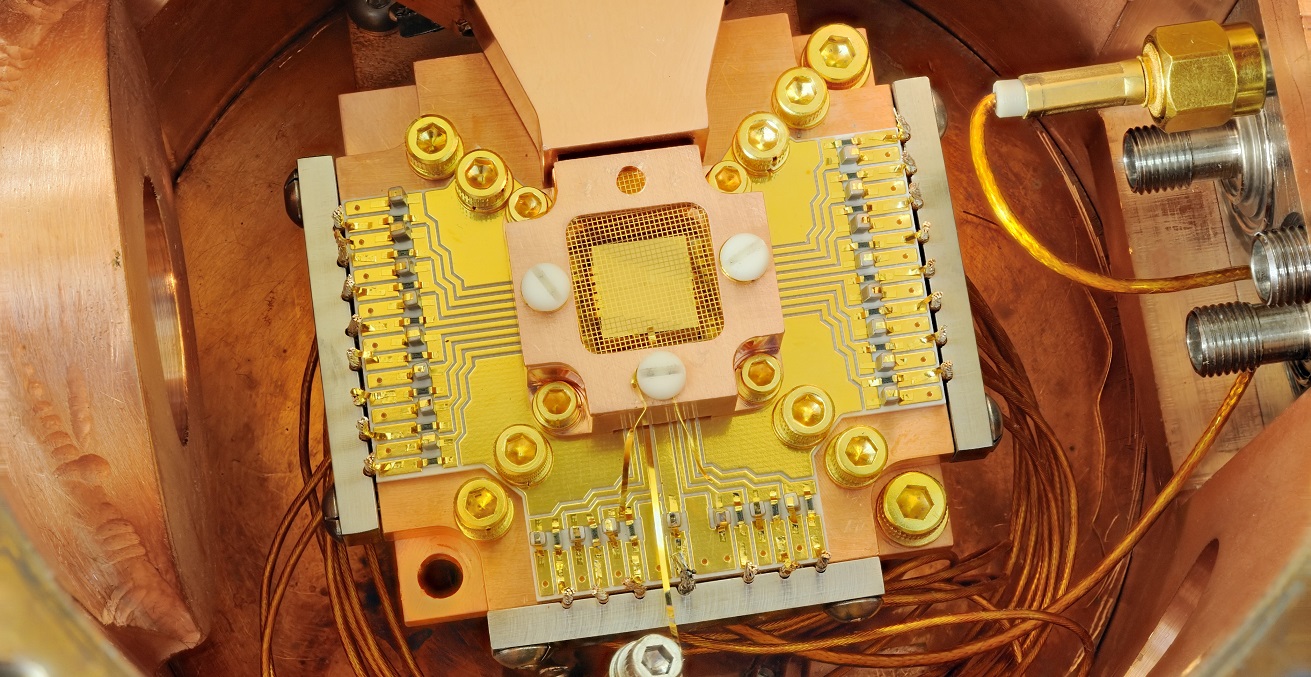
Most people have heard about quantum computing and know that it will be revolutionary. Few people, even government representatives, would be able to say exactly why.
While the details of what a quantum computer actually is are quite technical, the important thing to know is what a quantum computer can do, especially relative to a classical computer such as the one in the device on which you are reading this article. Although there have been some good attempts to explain how quantum computers work for the general public, these still largely miss the point. For example, Justin Trudeau gave a good description of qubits (quantum bits – similar to the ones and zeros in a classical computer), but he implies that quantum computing will make computers smaller, which is not particularly relevant. Since the era of room-sized computers, the drive for increasingly smaller computers, to the point where they are now microscopic, has made processors more powerful and computing less energy-intensive. As quantum computers are unlikely to be viable for consumer use in the near future, their size is not really a matter of concern. The two key reasons governments are investing in developing quantum computing technologies are quantum cryptography and quantum simulation.
Quantum cryptography has important applications in the distribution of secure information, which has garnered the attention of governments and militaries around the world. The objective of quantum cryptography is to mitigate the potential problems of sharing secret information between two parties, such as message interception or reliance human components of information sharing networks. Important applications for quantum cryptography are securing information sent over the internet and the distribution of nuclear authentication codes.
In the nuclear launch process, “nuclear codes” are used to establish the identity of the party issuing the launch command. This is achieved by producing an encryption key and dividing it into two parts. One part is distributed to the president, and the other is given to the launch sites. The distribution of these codes to the launch sites, currently done manually, is a potential weak point in this system. If another party were to intercept the encryption key and replace the nuclear codes with incorrect codes, then they will have disabled the US’s nuclear launch capability. This kind of attack is referred to as a “man in the middle” attack.
An interesting feature of quantum cryptography is that it offers a method of distribution that can detect digital eavesdropping and interference. This would allow for the distribution of nuclear codes to take place via a quantum network, where the integrity of the network and authenticity of the message can be ensured by quantum cryptography. The promise of a vulnerability-free channel to disseminate nuclear codes has made quantum cryptography an important avenue of research for defence departments in the US and China.
A much less significant reason for interest in quantum cryptography, which is often the focus of the media, is the ability to efficiently “crack” standard public key encryption methods. The secure transfer of information over the internet underpins many of important day-to-day tasks, such as internet banking and entering a password to login to a website. Encryption prevents the numerous third parties that handle that internet traffic from reading personal secure information. Currently, encryption is based on “public key” methods and relies on the difficulty in factorising numbers into their prime factors. Specifically, the algorithms are built around sub-prime numbers, which are the results of two very large prime numbers multiplied together. Prime factorisation an ideal problem because it is very difficult to find the prime factors, but very easy to check if those two numbers multiplied together equal the subprime number.
When the subprime numbers used to encrypt data increase, the computing power required to crack them increases exponentially. While it is unlikely that an algorithm run on a classical computer exists that can solve this kind of problem within a reasonable timeframe, quantum algorithms exist that could feasibly “crack” this encryption in a short time period. However, running these algorithms still requires a sufficiently large quantum computer. While a large quantum computer would be quite small, this capability is still well beyond today’s quantum computer demonstrations. For this reason, quantum code cracking seems unlikely to manifest in the near future.
Quantum simulation is the most far-reaching application for quantum computing, with important applications in chemistry, pharmaceuticals, medicine, and materials science. In particular, the efficient simulation of large molecular systems and reactions has massive implications for designing pharmaceuticals. Quantum simulation makes it possible to verify the effectiveness of new drugs or materials quickly and cheaply because it eliminates the need to synthesise a physical product for testing. Traditionally, new drugs take years to develop and pass through clinical trials. With a quantum computer, the accurate simulation of thousands of molecular arrangements can be tested as candidates for a new drug in a matter of hours or days.
When attempting these simulations on a classical computer, the computing resources required grow exponentially with the complexity of the molecule. Even for a simple molecular system such as caffeine (C8H10N4O2), the amount of classical computer memory required to model it can quickly exceed all the matter on earth. A slightly bigger molecule, such as penicillin (C16H18N2O4S), would require all the matter in the visible universe. A protein such as haemoglobin (C2952H4664O832N812S8Fe4) is thus comparatively gigantic and decidedly impossible to simulate on a classical computer. Fundamentally, this is a problem of trying to represent a quantum system using classical memory. If, instead, a quantum computer is used to represent and simulate the molecule, exponentially fewer information processing operations are required to achieve its result.
All of these high-impact applications mean that control and leadership of quantum computing technology will have significant economic implications over the coming decades. Quantum computing offers an opportunity to dramatically increase the scientific capacities of nations. It is likely that a “Quantum Silicon Valley” will emerge as the best minds in the industry seek the company of one another. Given the current lurch toward isolationism, it may be that a country must either be in the quantum race now and reap the benefits later or be behind for decades to come.
While the quantum encryption and the breaking of public key encryptions have seen the greatest interest from a security centric view and have garnered a great deal of government attention, the exciting near-term possibilities of quantum technologies lie in quantum simulation. With a likely year-long wait for a viable vaccine to emerge from the oversaturated field of candidates to ease the coronavirus pandemic, the potential nuclear security benefits of quantum technology may pale in comparison to the number of lives that can be saved with quantum simulation.
Alexander Ratcliffe is a PhD candidate in the Department of Quantum Science at the Australian National University. His research focuses on developing control schemes for scalable quantum information processing architectures.
This article is published under a Creative Commons Licence and may be republished with attribution.